Molecular Cardiology
Explore the molecular cardiology research programs and labs below.
Hossein Ardehali, MD, PhDStudying the role of mitochondria and metabolism in cancer growth, cardiac disease and immune processes, focusing on the role of hexokinases in immune function and stem cell differentiation, characterization of cellular and mitochondrial iron regulation and the role of mRNA-binding proteins in metabolism.
Studying the role of mitochondria and metabolism in cancer growth, cardiac disease and immune processes, focusing on the role of hexokinases in immune function and stem cell differentiation, characterization of cellular and mitochondrial iron regulation and the role of mRNA-binding proteins in metabolism.
Innovation is the key factor that drives science. Our lab has been at FCVRRI at Northwestern since 2005. We use various techniques, including various mouse models and in vitro studies such as tissue culture, hiPSC, CRISPR-Cas9, medicinal chemistry, heart perfusion studies, cancer models, hepatocyte isolation and immunological assays.
Ongoing projects in the lab include:
- Role of hexokinase binding to the mitochondria in cellular function
- Role of mRNA-binding proteins in cellular metabolism, mitochondrial function, and diabetes
- Mechanisms of cellular and systemic iron homeostasis and the effects of iron on epigenetics
- Role of Zpf36l1 and Zfp36l2 in cardiogenesis and cardiac development
See the faculty profile for Hossein Ardehali, MD, PhD, or visit the Ardehali Lab site to learn more.
Rishi Arora, MDInvestigating the molecular mechanisms underlying heart rhythm disorders, particularly atrial fibrillation, using clinically relevant large animal models to study the pathophysiology of atrial fibrillation. We are heavily invested in discovering new, gene-based therapies for atrial fibrillation.
Investigating the molecular mechanisms underlying heart rhythm disorders, particularly atrial fibrillation, using clinically relevant large animal models to study the pathophysiology of atrial fibrillation. We are heavily invested in discovering new, gene-based therapies for atrial fibrillation.
The laboratory's focus is to understand the molecular mechanisms underlying heart rhythm disorders. The laboratory is primarily known for its work on the mechanisms of atrial fibrillation, the most common heart rhythm disorder. Atrial fibrillation affects >6 million American and is a major cause of stroke. The incidence of atrial fibrillation increases with age, making it an ‘epidemic’ in an aging population. The management of atrial fibrillation is therefore an important aspect of cardiovascular medicine.
The laboratory performs the entire length and breadth of cardiac electrophysiology research. Techniques used include large animal in-vivo atrial mapping, high-resolution optical mapping, single cell electrophysiology, calcium cycling, gene-based targeting of signaling pathways in the intact large animal atrium and digital signal processing of atrial fibrillation electrograms.
Current Projects & Recent Work
- Role of the autonomic nervous system in atrial fibrillation
- Role of inflammation and oxidative injury in atrial fibrillation
- Gene-based targeting of oxidative injury, parasympathetic signaling and pro-fibrotic signaling in atrial fibrillation
Lab Members
Shin Yoo, David Johnson, Wenwei Zhang, Markus Rottmann, Amy Burrell, Anna Pfenniger, Suman Mandaa, Vamsi Pisipati, Justin Ng, William Marszalec, Yeonsik Choi, Rishi Arora, MD (PI)
Contact Us
300 E. Superior St., Tarry 12
Chicago, IL 60611
r-arora@northwestern.edu
Paul Burridge, PhDInvestigating the pharmacogenomics of chemotherapy-induced toxicity and electrophysiological disorders using human induced pluripotent stem cells.
Investigating the pharmacogenomics of chemotherapy-induced toxicity and electrophysiological disorders using human induced pluripotent stem cells.
The Burridge Lab works in the fields of pharmacogenomics (precision medicine), cardio-oncology, cardiovascular disease modeling, regenerative medicine, cancer, and cultivated meat, using induced pluripotent stem cells (iPSCs) as our model.
We specialize in large-scale hiPSC projects that required reprogramming, sequencing, editing, differentiating, and phenotyping hundreds of hiPSC lines.
Ongoing projects include:
- Studying the role of the genome in complex disease traits, understanding how single genetic variants modulate drug efficacy and toxicity, predicting which patients will experience successful therapy or suffer adverse drug responses, and discovering new drugs to overcome these complications.
- Developing new tools to reprogram, culture, and differentiate iPSC in an efficient and cost-effective manner to improve the use, scale, and ubiquity of these across all areas of research.
- Studying the genomics of arrhythmia and sudden cardiac death and devising precision medicine-led drug discovery.
- The applications of iPSC and direct reprogramming in regenerative medicine, repairing the heart after a heart attack, or ameliorating the effects of heart failure.
- How heritable (germline) variation influences cancer progression, oncology drug efficacy, and acquisition of resistance.
- The applications of iPSC-derived cells for cultivated meat.
Contact Us
Searle 8-450 320 E. Superior St.
Chicago, IL 60611 United Statespaul.burridge@northwestern.edu
See the faculty profile for Paul W. Burridge, PhD, or visit the Burridge Lab website to learn more.
Mesut Eren, PhDStudying the molecular physiology of the plasminogen activation system.
Primarily, Eren is interested in investigating the role of plasminogen activator inhibitor type-1 (PAI-1) in senescence and aging of various tissues including cardiovascular, renal and pulmonary tissues as well as cardiac fibrosis, heart failure and hair loss.
In order to study how PAI-1 affects the molecular mechanisms of these pathophysiologies, we developed novel mouse models, including the mice line that expresses the identical loss-of-function (LOF) mutant form of PAI-1 present in Berne Amish kindred and the line that lacks extracellular PAI-1 activity. Furthermore, we developed new monoclonal antibodies to detect and quantify this LOF mutant form of PAI-1 to quantify and test if it has any physiological and/or pathophysiological roles. Additionally, we have generated mice lines that have PAI-1 deficiency in cardiomyocytes and endothelial cells to explore the transcriptomics and metabolimcs changes in cardiovascular aging, cardiac fibrosis and heart failure with preserved ejection fraction (HFpEF).
Moreover, we have started an novel project that involves studying the role of PAI-1 in age dependent hearing loss using our mouse models described above in addition to the transgenic mice that express a functionally stable variant of human PAI-1.
See the faculty profile for Mesut Eren, PhD, to learn more.
Al George, MDInvestigating the structure, function, pharmacology and molecular genetics of ion channels and channelopathies.
Ion channels are ubiquitous membrane proteins that serve a variety of important physiological functions, provide targets for many types of pharmacological agents and are encoded by genes that can be the basis for inherited diseases affecting the heart, skeletal muscle and nervous system.
Our research program is focused on the structure, function, pharmacology and molecular genetics of ion channels. We were the first to determine the functional consequences of a human cardiac sodium channel mutation associated with an inherited cardiac arrhythmia. The lab has elucidated the functional and molecular consequences of several brain sodium channel mutations that cause various familial epilepsies and an inherited form of migraine. These finding have motivated pharmacological studies designed to find compounds that suppress aberrant functional behaviors caused by mutations.
See the faculty profile for Al George, MD, to learn more.
Asish K. Ghosh, PhD, FAHAInvestigating the epigenetic and molecular bases of stress-induced accelerated cardio-renal fibrogenesis and air pollution-induced cardiopulmonary pathology: emphasis on developing translational therapies targeting p300 and PAI-1.
Investigating the epigenetic and molecular bases of stress-induced accelerated cardio-renal fibrogenesis and air pollution-induced cardiopulmonary pathology: emphasis on developing translational therapies targeting p300 and PAI-1.
See the faculty profile for Asish Ghosh, PhD, FAHA, to learn more.
Epigenetic Regulation of Cardio-Renal Fibrogenesis by Acetyltransferase p300: Therapeutic Efficacies of Small Molecule Inhibitors Targeting p300.
Fibrosis is a common, yet complex, clinical manifestation of a wide spectrum of diseases including hypertension and diabetes-induced heart and kidney failure. Fibrosis is a major cause of disease-related morbidity and mortality worldwide. Early and late fibrogenesis events include: increased inflammation, cytokine imbalance, myofibroblast differentiation and an excessive accumulation of disorganized extracellular matrix proteins in stressed or injured tissues that leads to loss of tissue elasticity and ultimately, organ failure. There is no effective therapy for established fibrosis.
Research in Ghosh’s lab focuses on understanding the role of acetyltransferase p300 (p300), a major epigenetic writer, in extracellular matrix protein collagen synthesis and organ fibrosis. Ghosh’s research program has demonstrated and described the following novel findings, which illustrate fibrogenesis as an epigenetic event:
- Epigenetic regulator p300 is essential for profibrogenic signal-induced elevated collagen synthesis, the major matrix protein contributing to both reactive and reparative fibrosis.
- The levels of p300 and specific acetylation of histones are significantly elevated in myofibroblasts and different fibrotic tissues derived from murine models and heart failure patients.
- Inhibition of p300 activity decreases histone acetylation and fibrogenic processes including cellular proliferation, migration, myofibroblast differentiation and collagen synthesis in cardiac and renal cells.
- Small molecule inhibitor of p300 reduces hypertension-induced pathological cardiac hypertrophy and cardio-renal fibrosis. The beneficial effects are independent of blood pressure regulation.
- Post treatment of hypertensive mice with p300-specific small molecule inhibitors reverse specific histone acetylation and halt the progression of hypertension-induced myofibroblast differentiation, cardiac hypertrophy, fibrosis and diastolic dysfunction, without reducing elevated blood pressure.
- Lowering the level, not complete depletion, of p300 activity may be an ideal therapeutic approach to control hypertension-induced cardio-renal fibrogenesis and associated pathologies.
We are currently working on:
- Investigating the impact of elevated acetyltransferase p300 in stressor-induced endothelial and cardiac fibroblast senescence, and accelerated cardiac aging using cellular, biochemical, immunocyto-chemical, immunohistochemical and omics approaches.
- Evaluating the therapeutic efficacies of novel small molecule inhibitors of acetyltransferase p300 in prevention and reversal of stress-induced cellular aging and underlying mechanisms.
Contribution of Plasminogen Activator Inhibitor-1 (PAI-1) to Air-Pollutant Particulate Matter (PM2.5)-Induced Cardiopulmonary Pathologies: Therapeutic Efficacy of a Novel Small Molecule Inhibitor Targeting PAI-1
Air pollution is a global threat to human health and rest of the animal kingdom. Exposure to air pollutants like particulate matter causes cardiopulmonary and vascular diseases including pulmonary inflammation, vascular dysfunction, thrombosis, hypertension, arteriosclerosis, arrhythmias, and myocardial infarction. Exposure to air pollutant significantly increases the level of PAI-1, a secretory protein and most potent inhibitor t-PA/uPA serine proteases, regulates the tissue fibrinolytic system, and its deregulation contributes to cellular senescence/aging and different diseases, including vascular and cardiopulmonary diseases.
Recent findings on this project include:
- 5 induces the plasma levels of inflammatory, prothrombotic and profibrogenic PAI-1 in mice.
- Pharmacological inhibition of PAI-1 reduces the level of PM2.5-induced inflammatory markers in murine plasma, BAL fluid, lung and cardiac tissues.
- PAI-1 inhibition is protective against PM2.5-induced hypertension, cardiac hypertrophy and vascular thrombosis in mice.
- PAI-1 is a prospective druggable target for therapy of PM2.5-induced cardiopulmonary pathologies in humans.
We are currently working to understand in-depth the molecular bases of PM2.5-induced cardiopulmonary pathologies and protective effect of PAI-1 inhibition on PM2.5-induced cardiopulmonary pathologies, we are analyzing genome-wide pulmonary and cardiac transcriptome by RNA sequencing, bioinformatics and biochemical analysis.
Songwang Hou, PhDStudying phospholidpid functions in cells and anti-phosphatidylethanolamine antibody-resulted diseases in patients.
Hou's current research focus on two main aspects. One is the basic research of phospholidpid functions in cells, which includes their localization in membrane networks, the mechanisms of controlling phospholipid asymmetry and the pathological effects upon the defects. The another is the mechanism research of anti-phosphatidylethanolamine antibody resulted diseases in patients.
See the faculty profile of Songwang Hou, PhD, to learn more.
Sadiya S. Khan, MD, MScExamining the epidemology of and risk for heart failure.
Our studies include population-based cohorts, large electronic health record data analysis, and -OMICS (epigenomics, genomics, and proteomics) to perform mechanistic studies to enhance risk prediction and identify novel therapeutic agents for prevention and treatment approaches.
See the faculty profile for Sadiya S. Khan, MD, MSc, to learn more.
Francis Klocke, MDFocusing on coronary circulation and cardiac magnetic resonance imaging.
See the faculty profile for Francis Klocke, MD, to learn more.
Tsutomu Kume, PhDFocused on cardiovascular development and disease with an emphasis on the formation of the vascular system that is composed of blood and lymphatic vessels; we study the cellular and molecular mechanisms that control neovascularization in physiological and pathological processes using various mouse models.
Focused on cardiovascular development and disease with an emphasis on the formation of the vascular system that is composed of blood and lymphatic vessels; we study the cellular and molecular mechanisms that control neovascularization in physiological and pathological processes using various mouse models.
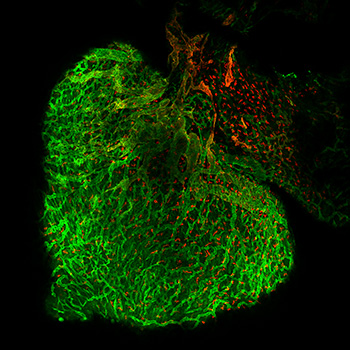
The Kume Laboratory is recognized for studies highlighting the role of a family of transcription factors termed Forkhead/Fox in diverse biological processes. Key observations include studies elucidating the role of Foxc1 and Foxc2 as (1) factors that serve as essential regulators of cardiovascular development, (2) key factors of angiogenesis and lymphangiogenesis, and (3) important regulators of the anterior eye segment. These research efforts have spawned novel areas of investigation with therapeutic implications for numerous biological systems, including the biology of the cardiovascular systems.
Studies in the lab have recently extended toward the area of the organ-specific vasculature, and we have established a research team to investigate mechanisms of tissue repair and regeneration using a variety of mouse models including ischemia-reperfusion injury.
See the faculty profile for Tsutomu Kume, PhD, to learn more. Daniel Lee, MDFocusing on both the technical development and clinical application of cardiovascular magnetic resonance imaging.
Lee's research laboratory focuses on both the technical development and clinical application of cardiovascular magnetic resonance imaging (CMR).
Viability Imaging
Initial research efforts focused on validating this technique’s ability to measure the extent of myocardial infarction and myocardial viability. Current investigations explore its use as a tool to measure, salvage, and predict remodeling following myocardial infarction, identify patients at risk for ventricular arrhythmias, and gauge the effect of experimental therapies on infarct size.
Myocardial Perfusion Imaging
Our research has demonstrated that first-pass magnetic resonance perfusion imaging has advantages over traditional radionuclide imaging for detecting modest reductions in myocardial blood flow and subtle differences between endocardial and epicardial flow. Investigators in our laboratory are currently developing techniques to measure absolute myocardial blood flow (in mL/min/g), which may allow more precise determination of blood flow limitations. These new techniques are and will be employed to characterize myocardial blood flow in patients with coronary artery disease, microvascular dysfunction, heart failure, and in studies of drug and stem cell therapy.
CMR Core Laboratory
CMR noninvasively obtains high-resolution images with excellent depiction of blood, infarct, and myocardial borders resulting in high reproducibility of measurements. Not surprisingly, CMR is increasingly being used in clinical trials to quantify left ventricular volumes, mass and infarct size.
See the faculty profile for Daniel C. Lee, MD, to learn more.
William Marszalec, PhDStudying molecular events underlying the development of experimental heart failure.
We have worked with the Northwestern ChemCore to develop a compound that we believe will inhibit the destruction of certain intracellular myocardial structures (t-tubules) that we belief to be an important prerequisite to heart failure. We have been testing the effects of over twenty such compounds on isolated myocytes and myocyte cell lines and have selected one agent that we are about to test in an in-vivo mouse model of heart failure.
Marszalec is also working to devise a method to make electrophysiological recordings from the cardiac autonomic ganglia and to ascertain the effects that released elements from adipose cells have upon them.
See the faculty profile for William Marszalec, PhD, to learn more.
Elizabeth M. McNally, MD, PhDIdentifying and studying mechanisms of mutations that cause genetic disease.
Mutations in cytoskeletal and sarcomere proteins cause heart failure and muscle weakness. We use novel computational methods to analyze whole genome sequencing from humans to identify novel genes and alleles linked to human disease. We model these mutations in human cells using induced pluripotent stem cell technologies to determine how these mutations act. We are defining gene-gene interactions using genome analysis, genome editing and quantitative trait locus mapping.
See the faculty profile for Elizabeth M. McNally, MD, PhD, or visit the McNally Lab website to learn more.
Ed Thorp, PhDInvestigating the cell and molecular biology of the cardiovascular system in steady state versus during inflammation.
Investigating the cell and molecular biology of the cardiovascular system in steady state versus during inflammation.
See the faculty profile for Ed Thorp, PhD, or visit Thorp Lab website to learn more.
Doug Vaughan, MDInvestigating the role of the plasminogen activator system in cardiovascular disease.
Investigating the role of the plasminogen activator system in cardiovascular disease.
Vaughan directs a multidisciplinary research group focused on investigating the role of the plasminogen activator system in cardiovascular disease. Active experimental programs are underway at the molecular and cellular level in animals and in humans. Transgenic and knockout mice are used in a variety of studies designed to explore the tissue-specific expression of PAI-1 in vivo and the role of the fibrinolytic system in vascular disease and tissue remodeling.
See the faculty profile for Douglas E. Vaughan, MD, to learn more.
J. Andrew Wasserstrom, PhDStudying how intracellular calcium cycling occurs in normal heart cells and how it changes during development of heart disease.
New evidence suggests that heart failure (HF) develops as the result of t-tubule remodeling in response to intracellular signaling during hypertension. The result is a change in intracellular Ca2+ cycling that underlies both diastolic and systolic dysfunction which eventually leads to HF. The lab investigates the molecular basis for t-tubule remodeling and how this leads to impaired Ca2+ cycling during the development of HF. The techniques currently in use in the lab include physiological measurements of intracellular Ca2+ cycling in isolated myocytes and in intact whole hearts using confocal microscopy, molecular techniques including RT- and QT-PCR, viral constructs and knock-out mice, biochemical techniques (Western blot) and immunohistochemistry in order to study the molecular and structural changes responsible for t-tubule loss and subsequent HF development.
A second project is investigating how a specific form of abnormal Ca2+ cycling found primarily in atrial myocytes, called triggered Ca2+ waves, might be responsible for the initiation of atrial fibrillation (AF). This novel form of intracellular Ca2+ cycling is characterized by propagated Ca2+ release events (waves) that occur only during rapid pacing and are absent immediately after pacing, distinguishing them from traditional spontaneous Ca2+ waves that occur exclusively during diastole. Interestingly, findings have indicated that triggered waves develop in tissues with little or no t-tubules including normal atrial myocytes and ventricular myocytes from failing hearts, suggesting an underlying structural basis for this form of potentially arrhythmogenic behavior. Most importantly, these triggered events are also more prevalent in atrial myocytes from failing hearts which could provide an explanation for why failing hearts are more susceptible to the development of AF than non-failing hearts.
Visit the faculty profile for J. Andrew Wasserstrom, PhD, to learn more. Lisa D. Wilsbacher, MD, PhDStudying the roles of G protein-coupled receptors in heart development and in the setting of adult heart disease.
Studying the roles of G protein-coupled receptors in heart development and in the setting of adult heart disease.
The goal of our research is to define key signaling pathways in cardiac development and cardiomyocyte maintenance in the setting of pathological stress. Currently, the laboratory investigates the G protein-coupled receptor sphingosine-1-phosphate receptor 1 (S1P1) and its unexpected role in cardiomyocyte proliferation and cardiac development. We aim to identify the signaling mechanisms that underlie these cardiac developmental effects and to investigate whether S1P1 signaling contributes to cardiac remodeling in the adult heart.
See the faculty profile for Lisa Wilsbacher, MD, PhD, and visit the Wilsbacher Lab site to learn more.
Shin Yoo, PhDWorking to discover significant physiological and pathophysiological phenomena and mechanisms by utilizing integrative and multidisciplinary approaches.
Yoo is currently working on a project about oxidative stress (OS) induced electrical remodeling in a large animal model of AF. AF is the most common arrhythmia in clinical practice, but the current therapies show suboptimal efficacy. Since the precise mechanisms by which OS causes AF are not known, Yoo discovered that OS likely contributes to electrical remodeling in AF by upregulating a constitutively active form of acetylcholine-dependent K+ current (IKAch) – called IKH - by a mechanism involving frequency-dependent activation of protein kinase C epsilon (PKCε). Furthermore, he showed that targeted expression of NOX2 shRNA in the intact atria of dogs subjected to rapid atrial pacing not only prevents the onset up to 12 weeks of follow up, ignificantly longer than any other gene-based approach to AF thus far but also the perpetuation of AF. This study seeks to not only shed light on some of the key molecular mechanisms underlying the genesis of AF but also proposes a highly novel, gene therapy approach to target these mechanisms. This study was recently published in Circulation (2020;142:1261).
See the faculty profile for Shin Yoo, PhD, to learn more.
Ming Zhao, PhDDeveloping diagnostic markers and investigates pathogenic mechanisms of human diseases based on changes in cellular membranes.
Major research areas in the Zhao Lab include:
- Apoptosis imaging technology development. Programmed cell death (apoptosis) plays a significant role in degenerative diseases. There is currently no clinical tool for assessing apoptosis in pathological conditions. Our research focuses on the development of optimal agents that combine sophisticated binding activities and favorable clearance kinetics for clinical translation.<.li>
- Assessing systemic toxicity in anticancer therapies. The outcome of chemotherapies hinges on the balance between tumor toxicity and patient tolerance. With the ability to noninvasively detect tissue apoptosis, we propose to assess anticancer therapies in a whole-body approach by monitoring tumor cell killing simultaneously with systemic tissue injury in response to chemotherapeutic agents. This is a transformative approach in oncology in terms of optimizing therapies on an individualized basis.
- Detecting myocardial injury in ischemic heart disease. Non-infarct myocardial injury in ischemic heart disease is of particular interest because this type of cardiac injury is not well understood in terms of its pathophysiological characteristics and its roles in long-term adverse cardiac events. Our research in this area focuses on the diagnosis of non-infarct myocardial injury, which in turn, will help address a significant gap in identifying patients at risk.
- Investigating the pathogenesis of antiphospholipid syndromes. The presence of circulating antibodies against phosphatidylethanolamine (PE) is positively correlated with clinical manifestations of antiphospholipid syndromes. However, the underlying pathogenic mechanism of anti-PE autoimmunity remains unknown. We have a major interest in investigating the cellular susceptibility to PE-binding agents, which in turn, will shed light on the potential pathogenic mechanism of aPE.
See the faculty profile for Ming Zhao, PhD, to learn more.